Water works
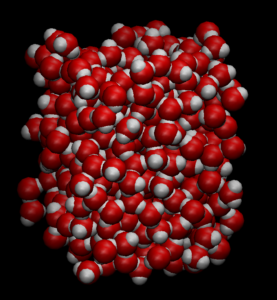
A section from a simulated liquid-water slab. Oxygen is red, hydrogen white. The water shows disorder in the hydrogen bonds, and the top surface is flat, suggesting that it fluctuates – it has ripples. (Image: Pacific Northwest National Laboratory.)
Water is ubiquitous and essential to life, but some of its traits can be surprisingly weird. Just ask Gregory Schenter, a chief scientist and laboratory fellow at the Department of Energy’s (DOE) Pacific Northwest National Laboratory (PNNL) who now leads a team probing its uncertainties at the atomic scale.
“Water is a unique molecular species,” Schenter says, “an odd duck.”
As the principal investigator of a DOE-supported molecular theory and modeling project, Schenter explores how water molecules behave in mixtures and other complex systems. In those cases, “some properties of water depend on an unknown balance between short-ranged and long-ranged forces. Sorting out all of this remains a challenge.” For example, researchers still don’t understand all the details of why oil and water don’t mix.
To explore that and other questions at the quantum scale, they’ve developed and used high-performance computer models. “We are pushing the field to do more complex systems,” he says.
Researchers have long been fascinated by water’s idiosyncrasies. For one thing, it and other similarly sized molecules should always form gases on Earth. Instead water can exist in our environment as a solid, liquid or gas. Water’s solid phase is unusual, too, because its density is lower than its liquid phase. As a result, ice cubes float in our beverages, and lakes and rivers freeze from the top down rather than the bottom up. Other unusual traits include water’s surface tension, which allows some insects to walk on it instead of drowning. Its capillary action allows columns of water solutions to stick to the sides of small vessels and tubes and even defy gravity to nourish plants.
Many of these features are caused by the special hydrogen bonds that form among interacting water molecules due to their structure and their balance of forces.
Each water molecule is bent, like a miniature boomerang. The central oxygen atom holds a slight negative charge, linked with two hydrogen atoms carrying a slight positive charge. The partial negative charge on water’s oxygen atom lets it attract the hydrogen atoms of other nearby water molecules. Those interactions between water molecules, called hydrogen bonds, are weaker than true molecular bonds. But they are still strong enough to organize water molecules into networks. These interactions constantly break and reassemble as the fluid flows and drips.
To completely account for water’s behavior, researchers also must consider other influences, Schenter says, such as van der Waals forces – the same phenomenon that allows geckos to walk up walls. Still other forces explain water’s remarkable ability to transfer heat.
“We know a lot about bulk, homogeneous water,” says Schenter, whose team is interested in exploring more complex systems and how those forces play out when water interacts with other types of molecules.
One example is how oil and water interact when mixed. When both liquids are poured into a glass, why do they separate rather than forming a single fluid?
The prevailing explanation is that the molecules are too fundamentally different to mix consistently. The hydrogen bonding between individual water molecules also makes them less likely to bond with oil molecules. Unlike water, oil molecules have very little separation of charges across their surfaces. For that reason, oil molecules and other chemicals that behave similarly are called hydrophobic, or water-avoiding. When mixed with water, they cluster among themselves, sometimes forming microscopic particles called micelles.
But when researchers zoom down to these driving forces at the atomic scale, groups like Schenter’s must grapple with quantum mechanics and fundamental physical uncertainty to understand exactly what’s going on.
At this quantum level researchers are still trying to understand exactly “how water flows, freezes, boils, controls chemical reactions and the synthesis and transformation of materials, and how water stores energy,” Schenter says. These types of studies are important to better understand the free energies of solvation – processes that direct how many ions can freely integrate into a water solution.
To decipher those processes, researchers first must simulate water alone. “Pure water is simpler than water with ions in it. It is a baseline,” Schenter explains. But “simpler” doesn’t mean easy. Computing simulations of less than 100 water molecules required millions of processor hours on Cori, a 30-petaflops high-performance computing system at the DOE’s Lawrence Berkeley National Laboratory.
In a June 2017 paper in The Journal of Chemical Physics Schenter’s group described simulations aimed at better understanding hydrophobicity and solvation. They used density functional theory (DFT), a suite of widely used methods for simulations in chemistry and materials science that incorporate quantum mechanics. DFT allows researchers to evaluate electron density around molecules relative to position.
Using a variation of DFT, the group simulated a cubical model of 256 water molecules coexisting and interacting under quantum rules at atmospheric pressure and 27 degrees Celsius (80 degrees Fahrenheit). The method simulated an atom-scale boundary between air and water that could be used, for example, to model surface tension. Though their evaluation revealed some potential uncertainties about that DFT variation, overall it provided “an excellent description of water at ambient conditions,” the authors concluded.
Future modeling studies will probe electrolytes, or “water with ions in it,” Schenter says. “We want to better understand and control how electrolytes change their properties as we change the amount and composition of the ions. The hydrogen-bonding network changes due to the presence of the dissolved solutes. Understanding this is the essence of the work.”
Schenter says he and colleagues are pushing to develop greater detail in their models. “The hypothesis is that subtle changes in this detail at interfaces of different forms of matter will significantly influence the forces between them and ultimately control the emergent phenomena.”
Once researchers understand the relation between various models, they can design a hierarchy of methods to span the problem’s range, Schenter says. “We are starting from a molecular scale and trying to understand how the details at this scale influence the properties at a macroscopic scale – a scale that we can see.”
About the Author
Monte Basgall is a freelance writer and former reporter for the Richmond Times-Dispatch, Miami Herald and Raleigh News & Observer. For 17 years he covered the basic sciences, engineering and environmental sciences at Duke University.