Revving up chemistry
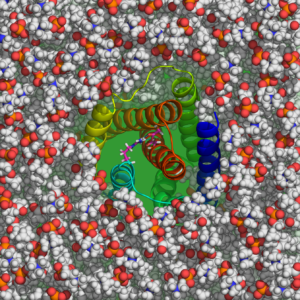
Understanding how plants tolerate droughts is a major chemical challenge that NWChemEx could help address. Above is shown the likely active site of a homolog of plant Bax inhibitor-1 protein, which controls the transport of calcium ions in the cells of sugarcane plants engineered to tolerate environmental stresses such as drought. The opening and closing of this channel (brightly colored ribbon structures) is controlled by hydrogen bonds between two residues (purple and blue). This channel is surrounded by membrane lipids (red, gray and white ball structures), which encapsulate plant cells and prevent molecules from moving back and forth. (Image: Hubertus van Dam & Qun Liu/Brookhaven National Laboratory.)
Even with today’s fastest supercomputers, scientists interested in simulating chemical reactions with high accuracy rapidly hit limits. Calculation costs balloon as chemists model more atoms, limiting them to examining smaller systems, comprised of fewer than a hundred atoms.
But as exascale computers – systems capable of a billion billion calculations per second – become available, new opportunities open to model complex molecular systems with unprecedented fidelity. How fuel molecules combust, how catalysts speed reactions, how plants tolerate droughts – exascale computing can address these and many other questions as never before.
Still, faster and better hardware isn’t enough to reach that goal. Computational chemists also need improved software and new algorithms to harness this increased power. As part of the Department of Energy’s Exascale Computing Project, researchers are addressing this challenge by redesigning and reimplementing NWChem, the field’s renowned open-source computational toolkit. A team developed NWChem in the 1990s for simulations on massively parallel computers. The new software, known as NWChemEx, supports the leap to exascale while boosting computational speed and efficiency on smaller terascale workstations and petascale servers.
This work echoes NWChem’s origin, which happened as hardware shifted from vector-based computing systems to those built using hundreds of less-powerful processors working in parallel. Jeff Nichols, now associate laboratory director for computing science at DOE’s Oak Ridge National Laboratory, led the project; Thom Dunning, a Battelle Fellow at DOE’s Pacific Northwest National Laboratory (PNNL) and a University of Washington research professor, oversaw the work and directs NWChemEx; and Robert Harrison of Stony Brook University was NWChem’s chief architect and serves in the same role for the new code.
“We’re at another point in history where computer technology is changing so much that we need essentially a new generation of chemistry software,” Harrison says.
Researchers have tweaked and optimized NWChem since its first release in 1997, scaling the code to hundreds of thousands of processor cores. But exascale computing poses new challenges. Today’s fastest supercomputers have heterogeneous hardware, a mixture of CPUs and GPUs (graphics processing units), new memory architectures and other innovative features.
The first exascale systems will most likely employ a similar CPU-GPU mix, but future machines might run on different hardware. NWChemEx’s design will maximize its performance on today’s HPC systems and adapt to hardware that hasn’t even been conceived yet. Says Dunning, “It’s bringing lots of new ideas into how you do dynamic load balancing,” distributing work evenly among processors, “and into how you separate the chemistry part of the code from the machine implementation itself.” Harrison adds that exascale isn’t only about building the first exascale computer but also “about the path of technology for the next 20 years.”
NWChem includes approximately four million lines of code, a massive piece of software. Theresa Windus of Iowa State University and the DOE’s Ames Laboratory is a longtime NWChem team member and NWChemEx’s deputy project director. She says the idea is to get the most “bang for the buck, so to speak, by writing code in such a way that if something changes in the hardware, we don’t have to change all the code.”
Windus, Dunning and Harrison have each worked on NWChem for more than 20 years, but a new generation of applied mathematicians, computer scientists and computational chemists is driving much of the day-to-day work on its successor. The teamwork is exciting, Windus says. “We’re looking forward to having this next-generation software with the next generation of computational scientists.”
The move to exascale also allows the team to work on longstanding challenges associated with algorithms and with scaling up highly accurate chemical computations, Dunning says. “We’re now up into the many tens of atoms, but these are still small molecules by chemistry standards.”
With current algorithms, increasing the size of a chemical system or boosting the accuracy comes with huge computational costs, Harrison points out. “Double the size of the molecule system, and our most accurate models require a factor of 128 increase in computing power. If you ask for one more digit in the accuracy, your cost just went up by a factor of 10,000.” To overcome those challenges, the team is considering reduced-scale algorithms – an approach that lets them lower the computational costs of these calculations dramatically, Windus says.
The NWChemEx project started in 2017, and the researchers spent much of their first year designing and prototyping their codes. This year they’ve ramped up their work on algorithms, implementing critical quantum-mechanical modeling methods. Building on this foundation, the team plans to have initial codes ready by the end of 2018. After that, the group will continue to optimize the code’s speed and efficiency. By 2020, the team aims for highly accurate calculations of molecules the size of ubiquitin, a protein with 1,231 atoms.
After that, the researchers will add additional capabilities to NWChemEx to model zeolites, cage-like catalytic structures that ensnare molecules and speed chemical reactions, including those that convert biomass feedstocks to fuels. “We have to be able to model the zeolite,” Dunning says, “and we have to be able to model all of the reactions that are going on in the zeolite.”
Understanding reactions’ speed and dynamic behavior hinges on how quickly chemicals attach to catalysts, react to form products and disengage from the catalysts. Molecules at real-world temperatures also rotate and vibrate on the atomic scale, so accurate calculations require sampling chemical systems repeatedly to account for these natural fluctuations.
More powerful chemistry simulations also could revolutionize how researchers examine the complexities of fuel combustion, in research such as the Pele project led by Jacqueline Chen at Sandia National Laboratories, Dunning adds. Combustion includes a complex mix of myriad molecules reacting quickly and under high heat and pressure, making it difficult to study in the laboratory. With more efficient computation, researchers could estimate important details such as the rates and energy input and output for important reactions in these systems.
Ultimately, exascale could support a huge leap forward for chemistry, Harrison notes. If NWChemEx and exascale computers can achieve fully predictive, chemically accurate calculations on complex systems, it “will transform our ability to design lots of new things. You could be talking about new processes for green energy production, new photovoltaic systems. The list is endless.”
Article Title
article text
About the Author
Sarah Webb is science media editor at the Krell Institute. She’s managing editor of DEIXIS: The DOE CSGF Annual and producer-host of the podcast Science in Parallel. She holds a Ph.D. in chemistry, a bachelor’s degree in German and completed a Fulbright fellowship doing organic chemistry research in Germany.