No passing zone
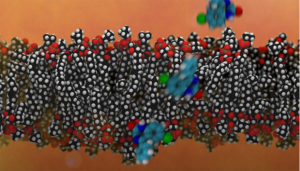
Drug molecules (cyan) pass through a cell membrane that simulates the blood-brain barrier. (Composite graphic: Tim Carpenter/Lawrence Livermore National Laboratory.)
Stroke, Alzheimer’s disease and many other medical conditions that attack the brain frustrate scientists who develop drugs to counter these life-altering and often fatal conditions.
Many new laboratory-crafted compounds trigger potentially beneficial effects on isolated cells but ultimately disappoint simply because not enough drug moves out of the blood stream and into the brain. The compounds cannot cross the blood-brain barrier, a layer of cells lining the brain capillaries. The barrier prevents germs and toxins from entering the body’s most vital organ but also blocks the entry of promising medications.
“If the drug can’t actually cross the blood-brain barrier to get into the brain, then when you come to doing some animal trials or human trials, you’ll find that the drug won’t work very well,” says Tim Carpenter, a computational biophysicist at the Department of Energy’s (DOE) Lawrence Livermore National Laboratory.
Drugs that cross the barrier could advance treatment of many other serious conditions besides stroke and Alzheimer’s disease, including brain or spinal cord injury, autism, ALS, AIDS and Huntington’s disease.
Researchers rely on costly and time-consuming laboratory experiments to see if a potential drug can reach the brain. As part of Livermore’s Biochemical and Biophysical Systems Group, led by Felice Lightstone, Carpenter has developed a computer model to examine barrier permeability. Simulations using the model agree with those experiments, and the group uses the calculations to check whether hypothetical compounds are permeable before they’re synthesized. The models help determine which potential drugs may be worth the effort and expense to develop further.
Funders include the DOE National Nuclear Security Administration (NNSA), Livermore’s internal lab-directed research program, the Livermore-led Biological Applications of Advanced Strategic Computing initiative and the military’s Defense Threat Reduction Agency.
Before Carpenter attacked the blood-brain barrier problem, he simulated brain-cell receptors. These proteins are embedded in the cell membranes and in everyday brain function they receive signals from neighboring cells in the form of molecules called neurotransmitters. Brain cell receptors also are the binding sites for medications that produce beneficial effects by mimicking, amplifying or interfering with neurotransmitters.
A particularly puzzling cell-membrane protein was GABA (neurotransmitter gamma-aminobutyric acid) type A (GABAAR), one of two receptor types that gather GABA signals. When GABA binds to GABAAR, it suppresses the cell’s activity. Because GABAAR plays this cell-calming role, it’s also the target of anti-anxiety medications, anesthetics and at least one potential chemical weapon.
To help make improved drugs, such as antidotes to possible weapons, Carpenter set out to learn how GABA binds to the receptor. The puzzle was challenging because GABAAR has a protein loop that covers the binding site like a lid.
Carpenter developed computer models of GABA and the receptor. He started with a molecular dynamics model, which represents each atom as a sphere, links the atoms together into molecules and allows the molecules to interact freely. He also coupled the molecular dynamics models with algorithms that enable each molecule to respond to known electrical charges and the energies inherent in movement and temperature. Using Livermore’s computer clusters Cab and Syrah, he ran simulations that placed GABA near GABAAR to watch their interactions.
But a neurotransmitter and its binding site do not always meet. In a realistic simulation that includes random motions, a neurotransmitter may wander away from its receptor as readily as it might head into binding range. Livermore has an advantage in tackling this, Carpenter says: Many of its computer clusters are configured to run multiple relatively small models. “Rather than running one long simulation and waiting for it to happen, we could run lots and lots of short ones and get some really good statistics on the interaction.”
He ran 100 short simulations. In 19 of them, GABA bound to GABAAR, revealing that the receptor has an electrostatic funnel that guides the neurotransmitter under the lid. The funnel is formed by a part of the receptor that has a strong negative charge “Once GABA got to that region, it was kind of passed along and ushered into the binding site,” Carpenter says.
These experiments led the team to a bigger problem, he says. “One of the next logical questions was, ‘OK, these are the properties that you need for a molecule to act on this receptor, but how does the molecule get there?’”
The team began to study how some compounds cross into the brain – and why others don’t. Clues came from existing medications. Pain relievers, antidepressants and antipsychotics cruise through the barrier, sidestepping the tight junctions between cells. Other drugs can’t (and shouldn’t) pass through. Treatments for the cardiovascular system, lungs, digestive tract and other parts of the body benefit targeted tissues without entering the brain, where they might cause harm.
Many drugs that cross the barrier do so by permeating cell membranes. They pass from the blood stream into barrier cells and out the other side, into the brain.
Carpenter built a computer model of a membrane to represent an individual blood-brain barrier cell. Like other cell membranes, it’s a lipid bilayer. Each lipid molecule has a polar head that’s attracted to water and two long, non-polar tails that are not. In water, the molecules self-assemble into a two-layered structure, with the hydrophilic heads facing the water and the hydrophobic tails facing one another.
“You need very specific properties to get your molecule across that barrier and into the brain,” Carpenter says. A non-polar molecule would not penetrate the polar outer surface, and a polar molecule could not mingle in the non-polar middle layer. Molecules that permeate the membrane have a mix of polar and non-polar properties. Like shape changers, they switch identities at will, blending into each crowd as they pass through.
Again using many short simulations, the Livermore team tested the cell-membrane model, using 12 different drugs with known barrier permeabilities. Molecular models of psychiatric medications crossed the barrier, as they do in patients and in animal models. Models of drugs that treat the heart, lungs, stomach and eyes were all rebuffed, as they are in the clinic and laboratory. That agreement with experiment established the model’s power to simulate hypothetical drugs.Carpenter and colleagues are expanding their research to ever more sophisticated cell-membrane models by including the effects of different lipid types. For example, they showed how some compounds work their way through the complex outer membrane of the potentially deadly bacterium Escherichia coli.
They are also studying how specific membrane lipids affect how proteins in those membranes function. “The membranes of cells can have hundreds of these different lipids in them, but they’re not uniformly mixed,” Carpenter says. “If you want to predict how these proteins are behaving, you first of all have to make sure that you have a realistic membrane environment.”
About the Author
Andy Boyles is a senior science writer at the Krell Institute and contributing science editor at Highlights for Children Inc.