Quantum designer
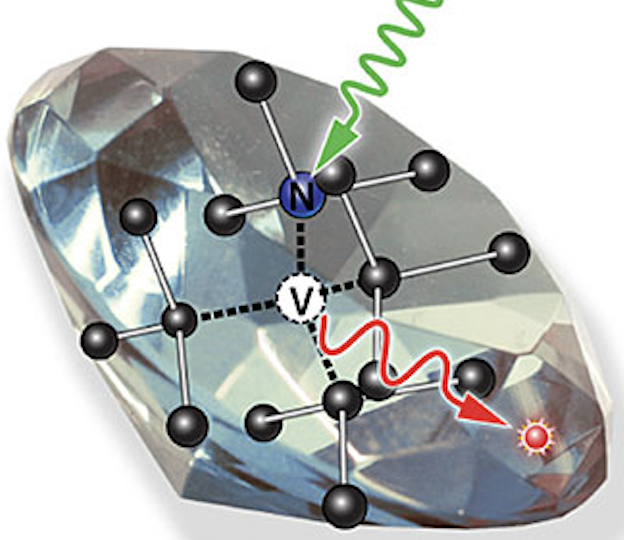
A photon emission from a nanodiamond’s single nitrogen vacancy center. (Illustration: Brookhaven National Laboratory.)
You could excuse Abigail Poteshman for taking a meandering path during her scientific career. While still in high school, she joined her first lab to conduct cancer cell experiments. Now, on the verge of earning her Ph.D. in computational and applied mathematics at the University of Chicago, Poteshman, a Department of Energy Computational Science Graduate Fellowship (DOE CSGF) recipient, is laser-focused on developing computer models for determining the optimal materials for quantum information and semiconductor technologies.
During that lab experience — an internship at Memorial Sloan Kettering Cancer Center in New York City — she met others using computation on the same problems but struggling to model the experimental findings.
“The conversations I had with the modeling folks really sparked an interest in the computer modeling side of research,” says Poteshman, who worked in the lab for three summers. “That was the beginning of my transition to computational work.”
As a University of Pennsylvania undergraduate studying physics and mathematics, she built models of electrons’ transitions between energy levels in different quantum systems. Though she was fascinated by the models, the lack of direct applications left her feeling unfulfilled.
When the time came to choose a doctoral research topic, Poteshman knew that she wanted to keep building computer models, but in collaboration with researchers who are working on real-world problems. She eventually decided on physicist Giulia Galli’s group. The lab focuses on deriving new ways to model quantum systems, with direct applications in sustainable energy, quantum technologies and microelectronics.
“Even though the group is predominantly chemists and physicists, Professor Galli was willing to take a chance on someone in an applied math program,” Poteshman says.
She’s designing materials for building quantum sensors and quantum information processing units — that is, qubits. She’s working with so-called spin defects in semiconductors, imperfections in a semiconductor’s crystal structure such as a missing atom or the insertion of a foreign atom. These defects can trap electrons, whose spin can be manipulated and used to store quantum information. The spin can be controlled with light and can hold information for a relatively long period of time, which could be useful for qubit design. The main challenge is that the defect’s spin can interact with those of nearby nuclei and lead to decoherence — the loss of stored information.
“Currently, there are no fast and efficient methods for analyzing these nuclear spins in materials with spin defects, as experimental characterization can be extremely time-consuming and labor-intensive,” Poteshman says.
Researchers can mitigate decoherence using dynamic decoupling, which involves applying quick, carefully timed microwave or laser pulses to a qubit to flip its spin, canceling out the effects of environmental noise over time. But optimizing the sequence and timing of these pulses is challenging. Although simulations can predict the success of dynamic decoupling under different conditions, the inverse problem of figuring out the interactions that occur during decoupling is complex.
Poteshman cracked this inverse problem using algorithms that iteratively analyzed data generated from computationally expensive electronic structure calculations performed by the Galli group. Poteshman validated her algorithms with experimental data provided by Argonne National Laboratory staff scientist F. Joseph Heremans, with whom Poteshman completed fellowship practicums in 2023 and 2024.
“We perform these really expensive computations with the idea that during the actual experiment,” Poteshman says, “we want to be able to offload as much computation as possible to yield a very fast calculation that an experimentalist could run on their laptop in the lab.”
Her method can be useful in designing better experiments and potentially lead to faster and more efficient characterization of defects in various semiconductor materials.
Along the way in 2022, Poteshman completed an additional practicum at Berkeley Lab with Jeffrey Neaton. She helped develop and implement a new algorithm to compute a quantity known as spontaneous polarization — a property useful for identifying materials known as ferroelectrics, which are used in technologies such as neuromorphic computers and tunable capacitors for wireless communications.
Besides helping Poteshman zero in on research problems she finds fulfilling, her practicums have provided another, unexpected benefit: “I got into running thanks to some friends I met.” She completed the Los Angeles and Chicago marathons in 2024. “I’m not very good, but I can show up and finish with a smile.”
Poteshman plans to seek a postdoctoral position in academia or industry after she graduates in 2026.
About the Author
Chris Palmer has written for more than two dozen publications, including Nature, The Scientist and Cancer Today and for the National Institutes of Health. He holds a doctorate in neuroscience from the University of Texas, Austin, and completed the Science Communication Program at the University of California, Santa Cruz.
You must be logged in to post a comment.